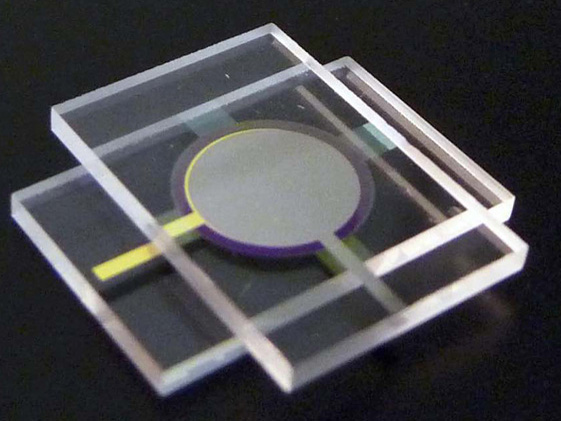
Nanofluidic capacitor
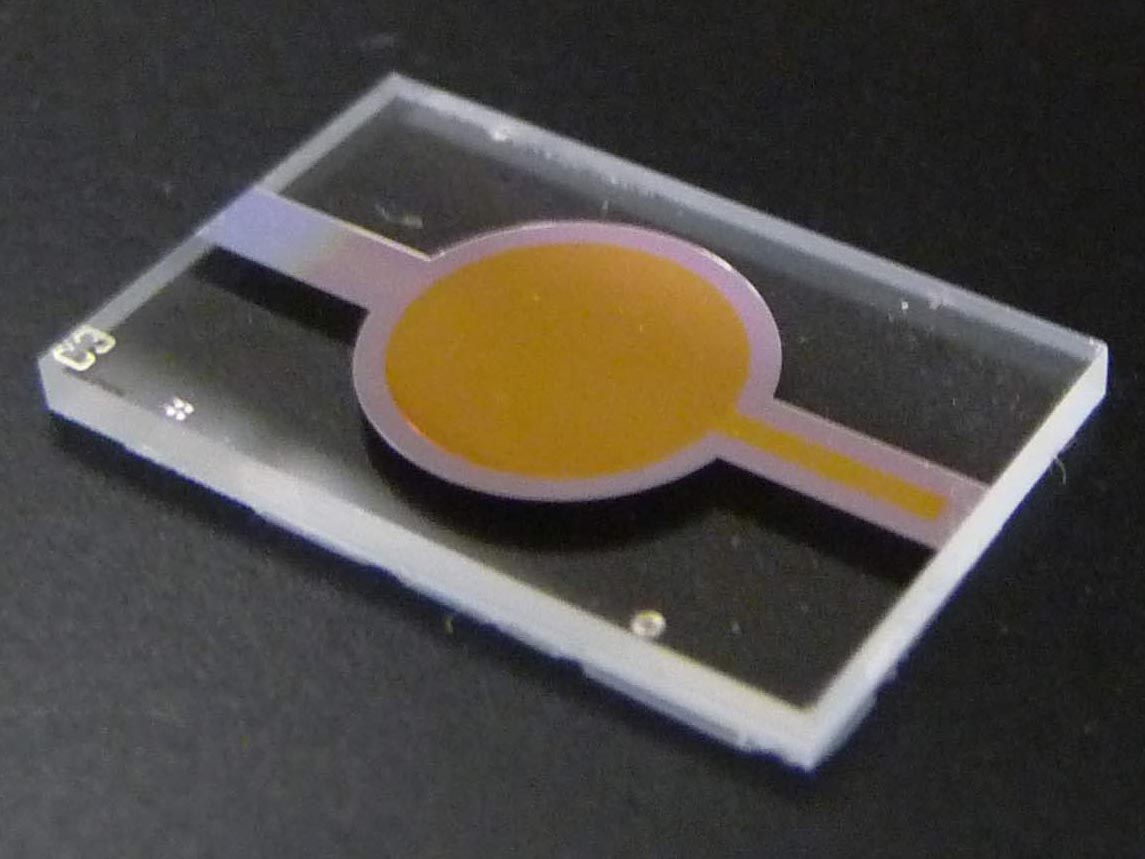
AlN nanofluidic piezoelectric transducer
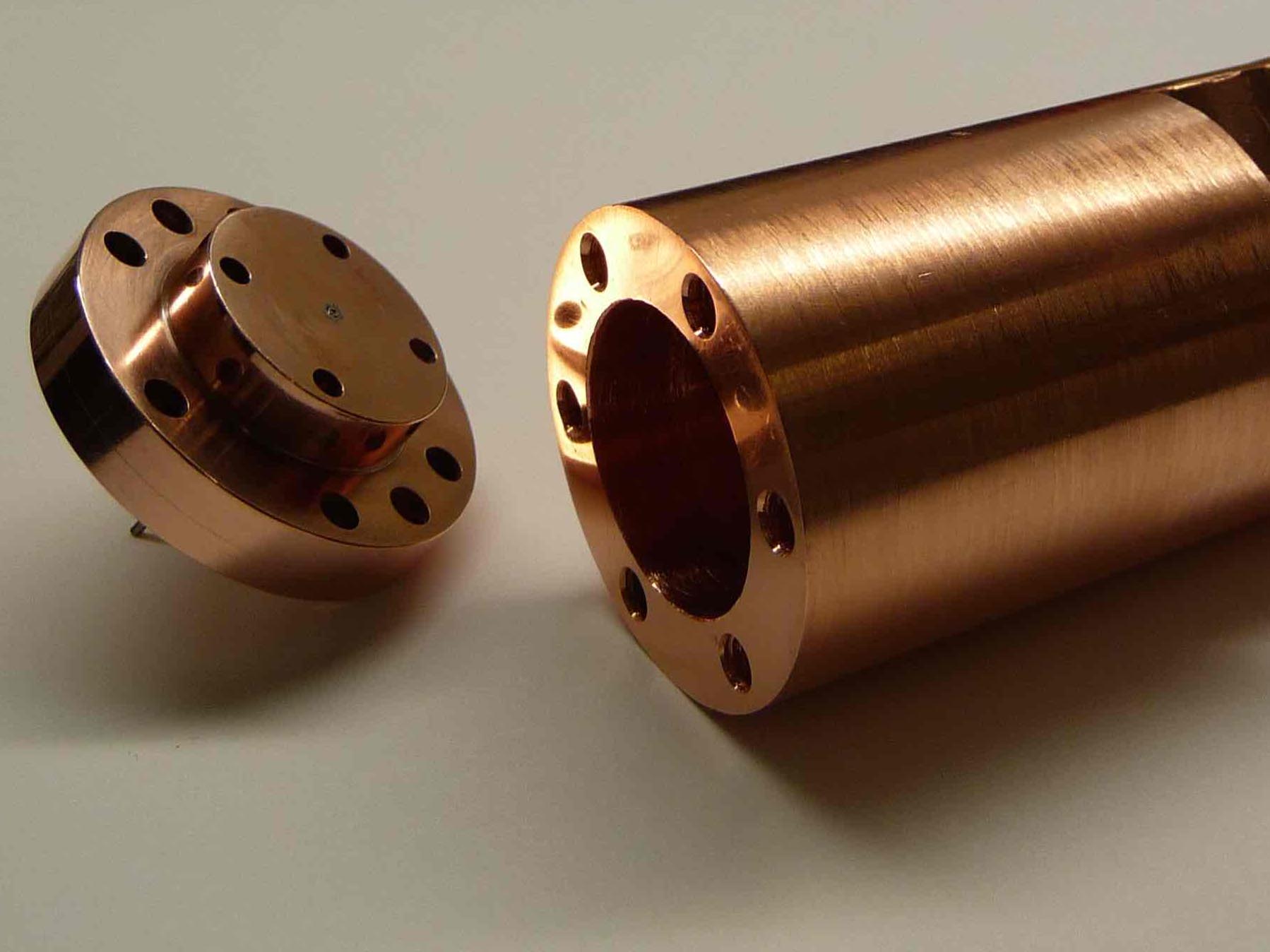
Copper sample cell
Description
In cavity optomechanics, the motion of a nanomechanical resonator is coupled to an optical or microwave cavity of high quality factor. Using this experimental setup, it is possible to optically detect the motion of a nanomechanical resonator with extremely high sensitivity, and even reach the ultimate limit imposed by the laws of quantum mechanics. Quantum-limited sensors are of great interest to many fields of research and applications. They can be used as highly sensitive detectors (e.g scanning microscopes, accelerometers, magnetometers).
In most cavity optomechanical systems, nanomechanical resonators are solid nanostructures (i.e. vibrating strings, beams, membranes or spheres) made of classical material (i.e. Si, Si3N4, SiO2). These mechanical resonators have intrinsic sources of energy dissipation that limit the sensitivity of the devices. In the quantum regime, since dissipation represents the coupling of the mechanical mode to the environment, lower dissipative mechanical systems have longer quantum coherence times and therefore have a potential for greatly improved quantum information processing. As a result, finding new materials with low dissipation, in particular for mechanical resonators operated at the quantum limit, is key to the progress of quantum optomechanics.
The major aspect of this programme is to exploit superfluid 4He, at low mK temperatures, as an ideal and flexible mechanical element for quantum optomechanics. A high quality factor acoustic superfluid cavity can be coupled both to a high quality factor superconducting microwave cavity. Recent progress in nanofabrication techniques allows the engineering of precisely defined structures, in which one or more dimensions can be reduced down to few tens of nanometers. Using these techniques, we will fabricate nanofluidic structures to confine superfluid 4He in order to generate superfluid acoustic resonances in nanoscale volumes. We will use a superconducting microwave cavity in order to detect the motion of our mechanical resonator with high sensitivity. This platform will allow us to undertake a versatile program of cavity optomechanics using superfluid based quantum mechanical systems in a regime beyond the limits of current theory.